1. Name of the location of 90% of epistaxis
2. A genetic disorder that forms AV malformations in the skin, lungs, brain etc
3. Name of posterior vascular plexus in the nasal cavity causing posterior epistaxis
4. 1st line treatment for all epistaxis
5. The common brand name for anterior nasal packing
6. Chemical used in cautery sticks
7. Physically scaring complication of posterior nasal packing with foleys catheter
Coming soon..
Vestibular Physiology for BSc Audiology Students
Remit
​
This page provides introductory information on vestibular physiology and the vestibulo-ocular reflex. It should be read in conjunction with the vestibular anatomy page.
Overview
​
When we think of balance we think of the inner ear. However, the inner ear is only one component of a more complex system that interacts to give us equilibrium. Look at the simplified flow chart below.
INPUT
PROCESSING
OUTPUT
Broadly speaking, information about our environment and our position within (derived from our senses) it is processed centrally (brainstem and cerebellum) and this processing results in an output. The output is usually a motor command (e.g. to an antigravity muscle or an eye muscle).
​
The inputs are:
​
-
Vision
-
Proprioception / somatosensation
-
Vestibular
​
Which is the most important? That depends; each is important in its own right and they are all contributing in some way all of the time. However, which we use most at any one time will vary according to our environment. For example, when you stand still on a firm, regular surface you are using your somatosensory / proprioceptive systems mostly. In this situations the semicircular canals and utricle are not contributing although the saccule is as it detects gravity when we are standing. You are able to close your eyes without falling and for the most part your vestibular system is not being stimulated. However, if you move to an unstable surface your vision becomes much more important.
Try it yourself
​
-
Stand on the floor with your eyes open and then closed. If you have a healthy balance system you will find that you are able to retain equilibrium with ease.
-
Next stand on a large cushion. Try the same exercise with your eyes open and closed. You will find that when you close your eyes you become unbalanced. It is best to do this with someone as an observer, in case you fall. On a sponge the somatosensory information is less reliable and the inner ear becomes more important as you sway.
Vestibular Inputs
​
Let's concentrate on the vestibular component of balance input. The membranous labyrinth is broadly divided into two functional regions: the part responsible for hearing (cochlea) and the part responsible for balance (utricle, saccule and three semicircular canals). This tutorial only looks at the latter.
​
The balance portion of the inner ear can be subdivided into areas that detect angular motion (pitch, yaw and roll) and areas that detect linear motion (front-to-back, side-to-side, and up-and-down).
​
There are balance organs on both sides of the head and these work together to provide accurate information for the purpose of static and dynamic balance. They are arranged in functional pairs and you will learn about these shortly. Before you do you will learn about the hair cell. This is the basic unit of all the neuroepithelia within the membranous labyrinth.
The Hair Cell
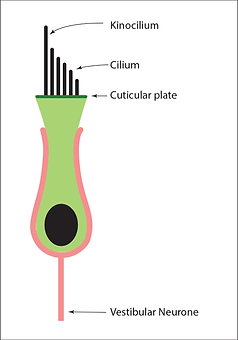
The diagram to the left represents a hair cell within the balance portion of the inner ear. It is just one of a large number of cells adjacent to one another that form the neuroepithelium. Remember that the neuroepithelia are found within the membranous labyrinth and are bathed in potassium rich endolymph.
​
Note that the cell has a tuft of 'cilia' at its apex and a single, longer kinocilium. The cell is intimately related to a nerve fibre (shown in pink).
​
Below is a magnified view of the 'cilia'.
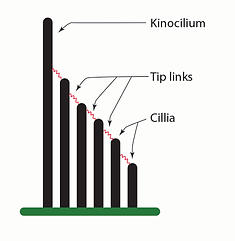
The tips of the 'cilia' are connected by links. When the 'cilia' move towards the kinocilium (not shown) these links are pulled and open channels allowing potassium from the endolymph surrounding the 'cilia' to flood into the cell. This causes depolarisation of the cell and an increase in its firing rate.
​
The channels are closed by myosin filaments that are activated by a concurrent increase in Calcium ions in the cell.
​
Note that the hair cell continually discharges even when the 'cilia' are stationary. Moving the 'cilia' only changes the rate of the firing - either by increasing it or decreasing it. The importance of this tonic discharge is discussed later.
Maculae and Cristae - Anatomy and Physiology
​
Next we will examine the anatomic arrangement of the hair cells within the maculae of the utricle and saccule, and the cristae within the semicircular canals. First the maculae.
Maculae
​
The utricle and saccule house the maculae. These are patches of neuroepithelium that are responsible for changing linear motion into neural activity that the brain can understand. First we shall see the anatomy of the maculae in greater detail and then discuss its physiology.
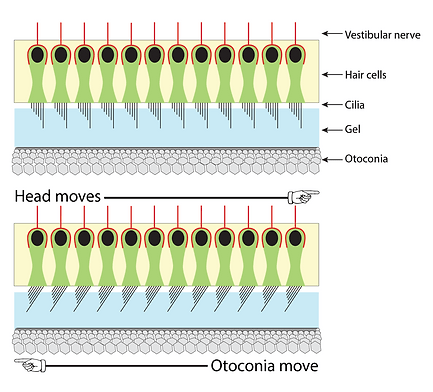
In this diagram the individual hair cells are shown in shades of green. Note that the tips of the 'cilia' and the kinocilium are embedded in a gel layer (blue).
​
Attached to the gel layer is a layer of otoconia. These are crystals of calcium carbonate and they give the gel layer mass and inertia.
When the head moves (top arrow) the gel layer lags behind by virtue of its inertia (bottom arrow).
​
This lag distorts the 'cilia' and a change in the firing rate of the nerve fibre results.
​
Remember that there is another macula on the opposite side of the head that is also being stimulated by this movement.
Cristae
​
Each of the semicircular canals has a dilation called the ampulla. The ampulla contains the crista - the organ responsible for detecting angular motion. First we shall see the anatomy of the crista in greater detail and then discuss its physiology.
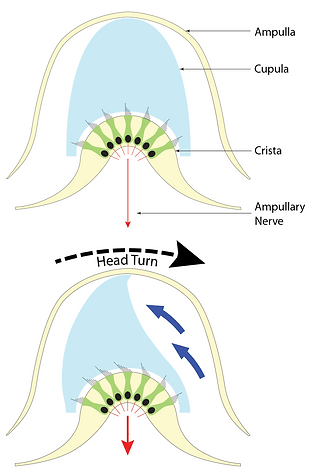
This is the dilation in the membranous labyrinth known as the ampulla. It contains a crista (Cr) and a cupula (Cu) made of gel (like in the macula but without the otoconia).
When the head turns (black arrow) the endolymph within the semicircular canal lags behind for a short while.
​
This lag exerts an oppositely directed force on the cupula. The bending of the cupula distorts the 'cilia' and this causes a change in the firing rate of the neuroepithelium.
​
There is another semicircular canal on the other side of the head that is influenced by the same head motion. Its neural output is affected also but as you will see in 'Functional Pairs' it's output will change in the opposite direction.
Functional Pairs
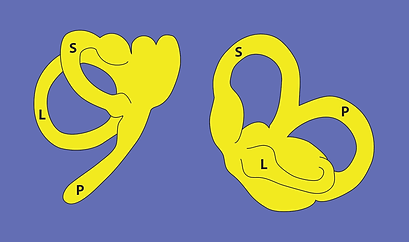
On the left there is a diagram of the left semicircular canals as viewed from superiorly and laterally. L, S and P label the lateral, superior and posterior semicircular canals respectively.
Notice that when viewed from laterally the lateral semicircular canal is seen not to be in a horizontal plane. In fact it is about 30° tilted backwards.
Looking from above at both sides, as in the next diagram, we can start to see some of the geometry of the semicircular canals.
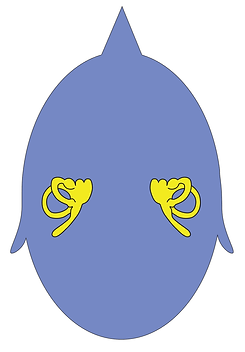
Look carefully at the lateral semicircular canals. Note that they are in the same plane as each other.
​
Now look at the left posterior semicircular canal. It is in the same plane as the right superior canal.
​
Likewise the left superior canal is in the same plane as the right posterior canal.
​
Each of these pairs is termed a functional pair. As you will see next, when one canal's neural activity increases because of a movement, the functional pair decreases its activity.
For ease of understanding lets look at the lateral semicircular canals only. In the diagram below we are looking down upon the two lateral canals - a functional pair.
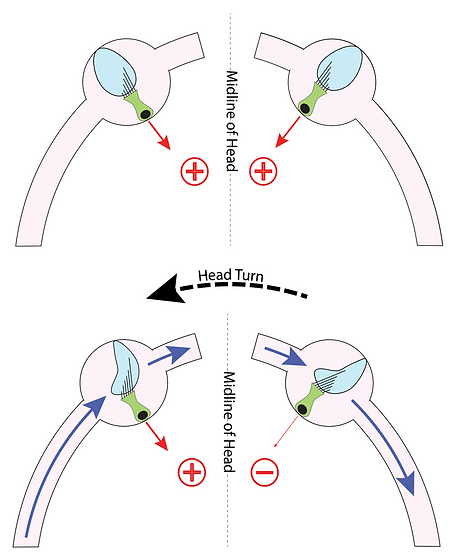
First note that the hair cells are arranged as mirror images: the kinocilium is always towards the midline in the lateral semicircular canals.
​
When the head turns to the left (curved black arrow) the fluids within the canal lag behind. This lag displaces the cupula within both ampullae at the same time (small blue arrows). But note: the displacement is towards the centre of the head on the left side and away from the centre of the head on the right.
​
The effect of this is that the neural activity in the left canal goes up while the neural activity in the right canal goes down.
​
In all functional pairs, regardless of their orientation, when a head movement raises the neural activity in one canal its functional pair will decrease its activity.
​
Functional pairing in the maculae
​
While the semicircular canals act as functional pairs across the head this is not true of the maculae. Instead the hair cells in the maculae are arranged in paired rows such that when activity in one of the rows goes up its paired row decreases activity.
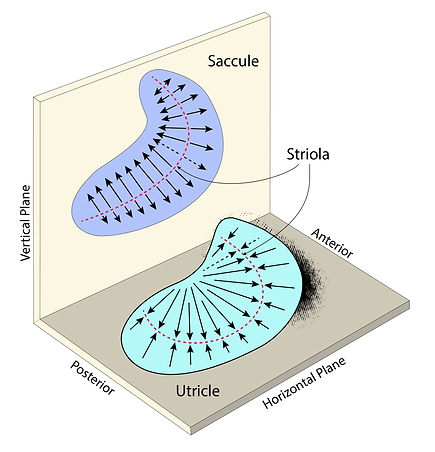
This diagram shows the approximate orientation of the maculae within the utricle and saccule.
​
Each macula has a line down the middle of it called the striola (red dotted lines). The arrowed lines represent a single paired row of hair cells. One pair is identified as a black dotted line in the diagram.
​
Along any given row pair the hair cells are all orientated in the same way. For example, in the utricle every hair cell has its kinocilium nearest to the striola. This means that the half of the row pair that is on the inside of the striola all align towards the striola as does the half of the pair that is on the outside of the striola.
​
Adjacent row pairs are not parallel with each other but fan out so that multiple planes in space can be represented. The dotted row in the utricle on this diagram will detect movement in an antero-posterior plane
​
Note that in the saccule, the arrows point away from the striola but that in the utricle they point towards the striola.
The next diagram shows this in more detail. It represents a single row pair on the macula of the utricle. Note carefully the orientation of the kinocillia with respect to the striola. Whichever side of the striola they are they always point towards the striola.

To make life slightly difficult the kinocillia in the saccular macula do the exact opposite. Their kinocillia are always pointing away from the striola.
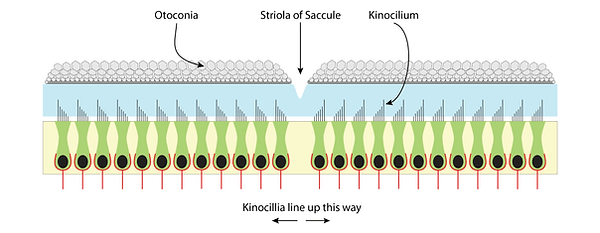
In the diagram opposite a single pair of hair cell rows have been highlighted to demonstrate how they act together during a given head movement.
​
In the utricle the kinocillia are lined up to point at the striola. Every hair cell in that row will be lined up in exactly the same way.
​
This means that the hair cells on the inside of the striola are pointing outwards while those hair cells on the outside of the striola are lined appointing inwards.
​
When the head moves in the direction of the black arrows the hair cells on the inside of the striola will be inhibited because their cilia will end away from their kinocillium. The hair cells on the outside of the striola will be stimulated because the cilia will be bending towards the kinocilia.

Remember:
​
​
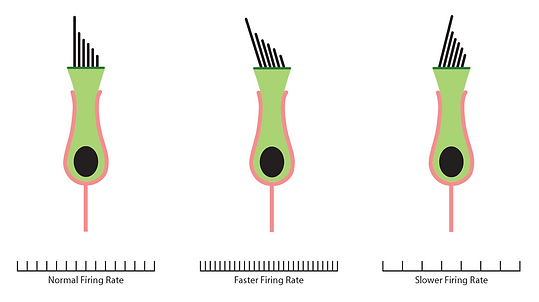
In this summary diagram the important facts from the previous discussion are drawn together. In it we see the hair cell. Remember that the hair cell is always causing firing of the nerve fibre at its base. This is the tonic firing rate. When the 'cilia' bend towards the kinocilium the firing rate increases and when they bend away the firing decreases.
​
Remember also that when a hair cell in a semicircular canal is bending towards the kinocilium on one side of the head the functionally paired hair cell on the other side of the head is decreasing its activity.
​
Functional pairing happens in the maculae too but the pairs aren't across the head from one another. instead they are within the same macula. Nonetheless, their equal and opposite firing rates work in the same way as those of the semicircular canals.
Paired Information and the Brain or 'why we sense vertigo'
​
Information in the form of neural impulses are sent via the vestibular nerves to the vestibular nuclei in the brain stem. Here it is integrated with other balance inputs to give us an accurate idea of where we are in space and what we must do to remain stable.
​
In order to understand why we sense a spinning sensation (vertigo) when we have an ear disease, let us consider a simplified view of what the brain does with the information it receives from the lateral semicircular canals.
​
For ease it is suggested that you consider the brain to do simple arithmetic with the information it receives. It compares the activity on one side with the activity on the other. If one side has more activity then the brain 'knows' that the head is turning towards that side. Look at the diagram below.
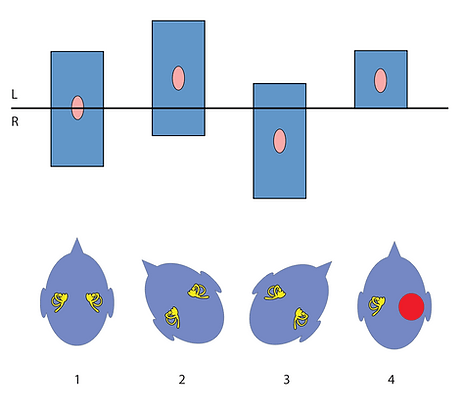
In this diagram the top half represents the brain's 'arithmetic'. The pink spots represent net activity of the two sides. Any net activity above the line represents left movement and any below the line right movement. L represents the degree of left neural firing from the left lateral canal and R represents neural firing from the right lateral canal. When the firing rate is equal on both sides the net activity is zero. To the brain this means no turning.
​
At the bottom we have four different scenarios. In 1 the subjects head is not moving. Both right and left lateral canals are producing the same rate of neural firing and the brain compares these. Because of the equality the brain knows that the head is not moving (pink spot on the line).
​
In 2 the subjects head is turning to the left. Left lateral canal activity goes up and right lateral activity goes down. Simply subtracting right from left activity tells the brain that there is net left activity and the brain knows that the head is moving to the left (pink spot to the left).
​
The same goes when the subject is turning to the right in 3. The right activity increases and the left activity decreases. Simple comparison tells the brain that there is net right activity and, therefore, that the head is turning to the right.
​
But what happens when the head is not moving and one of the lateral canals stops sending information to the brain? In diagram 4 the right ear stops sending information. The brain still compares left with right and finds that there is net left activity. It, therefore knows that the head has turned to the left - even though it has not really! Thus the brain tells the subject that there is turning when there is not. This is the sensation of vertigo.
Semicircular Canals and Eye Muscles
​
So now you know why we sense a spinning sensation when one inner ear stops working. Is it easy to understand where the nystagmus comes from? The answer is yes, but you first need to know how the semicircular canals are wired to the eyes.
​
The simple fact is that the majority of information provided by the semicircular canals is destined to go to the eye muscles. This is achieved in the brain stem by relays from the vestibular nuclei to the nuclei of the third, fourth and sixth cranial nerves. There are three (functional) pairs of semicircular canals and there are three pairs of extra-ocular muscles. This is no coincidence.
​
The next diagram is unnecessarily complex so only look at it briefly. We are looking down on the brainstem. It is not to scale.

It shows the connections between the three semicircular canals on the left and the three pairs of extra-ocular muscles. Note that only one ear is shown. The yellow muscles are the right lateral rectus and the left medial rectus. The occulo-motor nuclei are not shown nor are the inhibitory circuits.
​
Again it is easier to pick one pair of canals. Lets return to the lateral (horizontal) canals. The next diagram shows them both (the left is in yellow and the right in purple).

Look carefully at the two diagrams. On the left the head is stationary. Equal amounts of information are sent to all of the extra-ocular muscles and the eyes remain stationary.
In the right-side image, the head has turned to the left and the activity in the left lateral canal (LSCC) has increased (this is shown as an orange colour). This causes contraction of the muscles to which it is connected and the eyes turn to the right. At the same time the activity in the right lateral canal decreases (paler purple). This has the effect of decreasing the activity in the muscles to which it is connected. This assists in turning the eyes to the right because there is less resistance to movement.
The semicircular canals enable you to keep your eyes fixed on a target when your head is moving.
Test your lateral canals
​
Look at the 'X' below. Then quickly turn your head to one side while keeping your eyes on the 'X'. You will find that you can do this easily at most speeds. This is your lateral canals in action. They enable you to keep your eyes fixed on a target while your head is moving.
X
This ability is tremendously important. Without it our vision would be continually blurred unless we were absolutely still. In fact even when you are sitting perfectly still your head is bobbing up and down by a small amount. Do you know why? The answer is at the foot of the page.
​
​
The Lateral Canals and Nystagmus
​
We have seen the importance of the semicircular canals in keeping our vision stationary and how they react to movement of the head. What happens when one canal loses it's function through disease?
​
You should be able to work this out for yourselves. If you are finding it difficult look at the following diagrams.
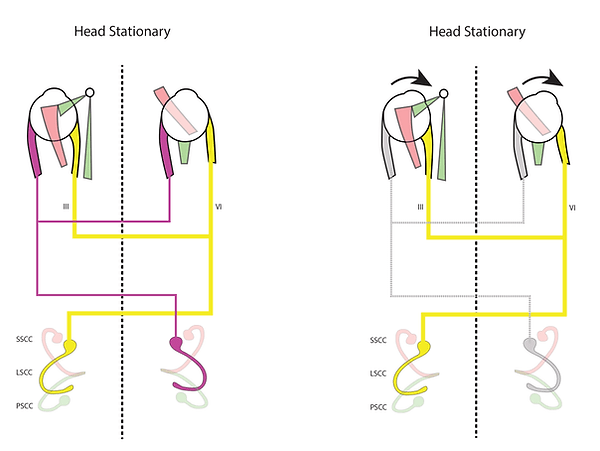
On the left the subject is not moving. There is equal firing from all canals and the muscles and eye remain stationary. But what happens when the right lateral canal stops functioning as normal (shown by pale grey and dotted lines)? The activity in the muscles to which it is attached drops. Now, even though there is no head motion, the eyes drift to the right through unopposed muscular action.
​
This slow drift from the midline is the first phase of jerk nystagmus. It is the pathologic phase. Shortly afterwards there is a sharp central correction for the erroneous drift. This is the fast phase of nystagmus. Recall that nystagmus is defined by its fast phase.
​
Thus, in ear disease, the nystagmus is directed away from the diseased ear.
​
These are the fundamentals of vestibular physiology. There is much more to it but that is beyond your syllabus. If you have any questions ask your tutor.
​
​
​
Answer: Your head is bobbing because of the force of blood pumping up your carotid arteries into your head.